Photothermal Microscopy
Non-fluorescent objects are difficult to detect optically once their size is considerably smaller than the optical wavelength and scattering cross sections are small. However, if they absorb light which is then subsequently converted into heat, refractive index changes in the local environment of the absorbing species can be detected. This type of spectroscopy is termed photothermal spectroscopy and allows access to non-fluorescent nano-objects even in the fundamental limit of a single quantum object.
The group explores several different subtopics with fundamental importance or as direct application of photothermal microscopy.
In detail the topics
- Hot Brownian Motion
- Light scattering of heated nanoparticles
- Twin-focus photothermal correlation spectroscopy
- Modeling and description of the PT signal
are of special interest to the group.
Theory of Photothermal Detection
A resonant heating laser (for AuNPs: 532 nm, surface plasmon resonance) with a wavelength that is absorbed by the species of interest as well as a non-resonant second probing laser (typically long wavelength, i.e. 635 nm) are both focused onto the same sample. The heating laser is modulated at a high frequency of about 200 kHz while the probing laser power transmitted through the sample and collected above is demodulated on the same frequency. The power absorbed by the NP and provided by the heating laser creates almost instantaneously a temperature profile around the particle (see image, red curve). Since the refractive index of the embedding material (water, cell-plasma, polymer) is temperature dependent a corresponding profile of the refractive index is thereby created (blue curve). The gradient of the refractive index thus generated with the frequency of the heating laser constitutes a thermal (divergent) lens. This lens affects the propagation of the probing laser and leads to a modulated transmitted power which is detected with a photodiode. Demodulation and normalization by the background detected power yields the rel. photothermal signal. The principle of photothermal detection can also be visualized with the PT signal simulator on our software page.
The typical experimental setup for PT microscopy is very similar to a regular confocal microscope. One microscope objective focuses the laser beams onto the sample while a second one collects the transmitted beams behind the sample (see image below). The transmitted light is then separated with a dichroic mirror and the sample can be scanned with the piezo-scanner onto which the sample is mounted. Typical collected intensities for such particle scans are shown in the image (red and green).
The corresponding PT signal is shown, too (red-blue image). The theory of the observable checker-board like patterns extends the generalized Lorenz Mie theory (GLMT) and has been developed over the past 2 years to perfectly match the observation (see published papers). The Mie-Theory is the framework of light-particle interaction (spherical in shape) which predicts rainbows and halos.
We have adapted the 100-year-old Mie-Theory for the purpose of this very special kind of light-particle interaction by the extension of the already generalized Mie theory (which was adapted by G. Gouesbet et al. to non-planar waves) to finite collection angle domains. This important extension then allows the calculation of the collected transmitted power after the light – particle/refractive index gradient interaction. The refractive index gradient (hot environment) around the particle has been included by the discretization into a finely layered sphere with the public c-code scatnlay.
A macroscopic experiment with a metal sphere embedded centrally in a transparent resin-cube nicely illustrates the thermal lens: When the metal sphere was heated with a 1 W green laser beam, an image taken from above shows the characteristic lens-like distortion. The animation shows a time-lapse of the effect (played inverse) while the sphere and the sample are cooling down.
Hot Brownian Motion
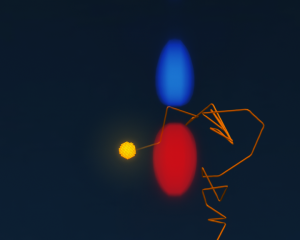
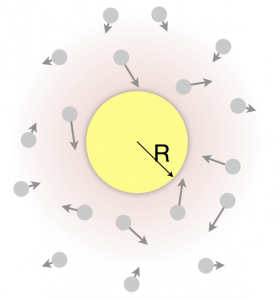
The meaning of temperature and viscosity, however, is not anymore obvious if the particle is heated and moves with a temperature and viscosity profile. The group studies this so-called “Hot Brownian Motion” with the help of photothermal correlation spectroscopy (see image: the trajectory of a AuNP through the split detection volume of TwinPhoCS is shown).
Light Scattering of Heated Nanoparticles
Photothermal microscopy involves the light scattering on a continuous refractive index gradient generated around the heated nano-object. We study in experiment and theory the light scattering of individual metal nanoparticles. The theoretical treatment involves a complex generalized Mie scattering of spherical refractive index gradients and metal-nanoparticles in focused aberrated laser beams. See the article “Photothermal Single-Particle Microscopy: Detection of a Nanolens” for details.
Twin-Focus Photothermal Correlation Spectroscopy
One of the direct outcomes of a rigorous theoretical treatment of light scattering from a refractive index gradient generated by a nanoparticle is that the refractive index gradient behaves like a nanolens. Focusing a probe laser on this divergent nanolens therefore results in either an increased or decreased photothermal signal depending on whether the focus of the detection laser is in front or behind the nanolens.
This splits the detection focus into two sharply separated regions. This allows for the detection of very weak axial motion of nanoparticles with the help of the photothermal correlation function. This high sensitivity to axial displacements is explored in biophysical applications.