In order to tackle the three fundamental research objectives we have devised a coordinated research programme covering three overlapping research areas: (A) chemical bonding, (B) membranes and diffusion and (C) labelling and detection. Each of the fourteen research projects is associated with one or more of these three research areas.
Research Objectives
For hydrogen isotopes, significant effects result from the quantum nature of matter. This leads to different discrete energy levels and hence different state distributions for the isotopologues. Quantum mechanical tunnel effects can be crucial and need to be considered. Among all elements, nuclear quantum effects are greatest for the hydrogen isotopes, owing to the largest relative isotope mass ratios. While the explanation of the trends observed for molecular properties of dihydrogen isotopologues is straight-forward, this is not the case anymore for a network of particles, and assessing the extent of the influence of nuclear quantum effects in bulk hydrogen is anything but trivial. This also holds true for the properties of water isotopologues, where a complex interplay of interactions governs the properties of hydrogen bond networks. This is highly attractive, because, in contrast to bulk matter, isolated clusters can be treated at high quantum chemistry levels, allowing to benchmark computational models and paving the way for obtaining a better fundamental understanding of NQE involving hydrogen isotopes.
Even though the large relative mass differences should allow for an efficient hydrogen isotope separation, current industrial technologies are energy-inefficient with a large potential for improvements. Nanotechnology has made the exploitation of nuclear quantum effects possible, but all of the proposed materials are subject to limitations that prevent wide-scale applications. Alternative separation approaches, working even at room temperature and in liquid phase, are based on isotope effects of the penetration barrier through 2D crystals and the entry barrier to the interstitial space in layered materials. However, these promising alternatives are in their infancy: their performance is far from being at a level where fundamental research can be succeeded by technological development, but most importantly, the underlying mechanisms are not well-understood yet. Thus, the second objective of 1,2,3H is to develop new hydrogen isotope separation materials that will be explicitly tested for H/D separation and, for selected, promising candidates, for T capture from radioactive waste. For the rational design of separation materials, we will combine the results that have been obtained in research areas A and B, and use the detection techniques developed in research area C to validate the performance of the proposed materials.
The third objective of 1,2,3H is to identify novel routes for the efficient synthesis of D- and T-containing drug-like molecules, e.g. by using photocatalysis and electrochemistry. Direct, late-stage hydrogen isotope exchange constitutes a powerful alternative to classical labelling strategies involving noble-metal catalysts or strong acids and bases and offers the promise of rapid exploration of structure-activity relationships, the blocking of metabolic hot spots and the preparation of biological probes. We will explore the possibility of selective capture of water isotopologues as well as selective bond activation using supramolecular hydrogen-bonded assemblies. To improve the performance and make use of flow-dependent advantages, we propose to perform the photocatalytic reactions in microflow systems.
Research Areas
Hydrogen isotope effects on chemical bonding
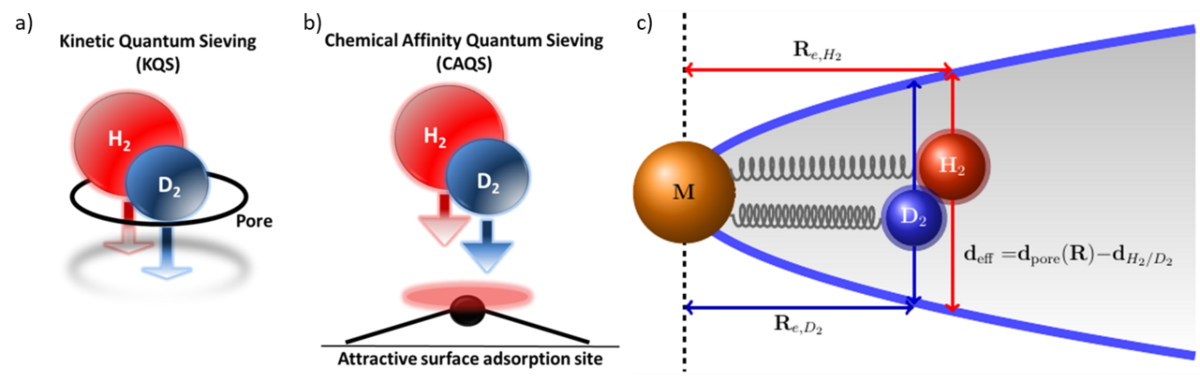
Objective: To understand how NQEs affect the chemical bonding of dihydrogen isotopologues, covalently bound hydrogen and hydrogen-bonded networks. To identify situations in which the isotope effect is sufficient for isotope separation.
Methodology: We combine spectroscopic investigations on molecules, clusters and nanoparticles with theoretical analysis. Theory: highly accurate structure and energy calculations while considering nuclear quantum effects, quantum-mechanical model calculations, topological analysis of electron density, energy decomposition analysis, simulation of EPR, IR and Raman spectra, identification of inelastic neutron scattering signals. Experiment: ion vibrational spectroscopy, single nanoparticle mass spectrometry, low temperature IR/Raman, EPR, inelastic neutron scattering (in collaboration with Oak Ridge National Laboratory).
Compounds: clusters, molecules, MOFs, zeolites.
Associated courses: BC2, BC4, AC1.
Research projects: P1, P2, P3, P4, P5, P6, P10, P13, P14
Nuclear quantum effects in hydrogen diffusion
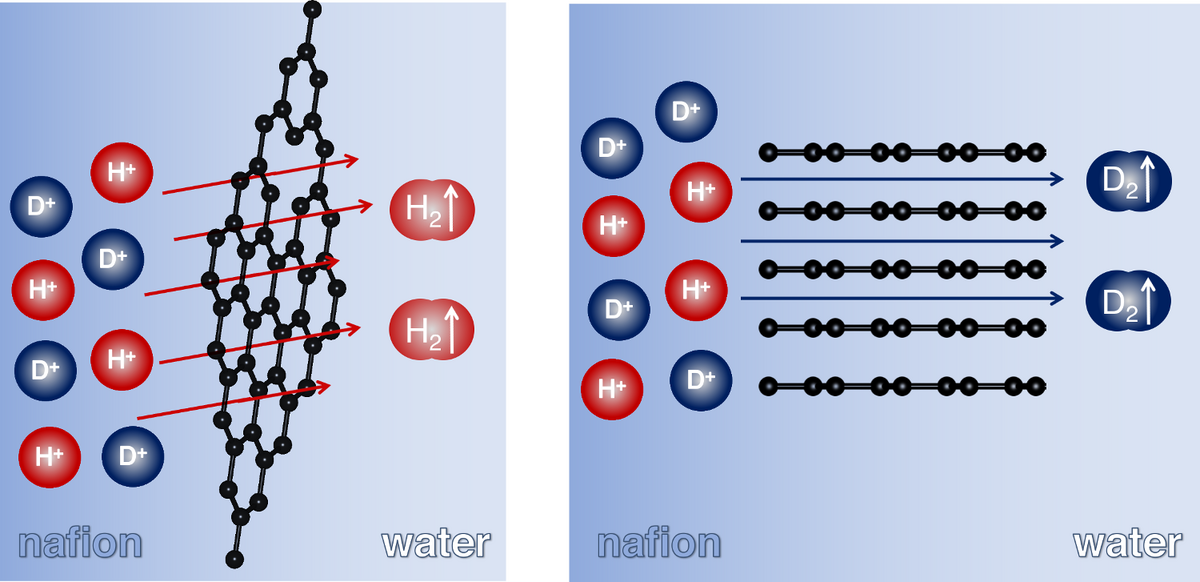
Objective: Quantitative understanding of nuclear quantum effects on diffusion and penetration barriers of hydrogen into cavities, pores and gates.
Methodology: We combine molecular simulation techniques that consider nuclear quantum effects, such as path-integral molecular dynamics simulations and kinetic Monte Carlo simulations with diffusion experiments, e.g. breakthrough measurements on upscaled materials using pulsed-field gradient nuclear magnetic resonance, detection of hydrogen penetration through 2D crystals and layered materials.
Compounds: atomically thin membranes of 2D crystals, layered materials, zeolites, MOFs.
Associated courses: BC2, BC4, AC1, AC3.
Research projects: P2, P3, P4, P6, P7, P8, P12.
Hydrogen isotope labelling and detection

Objective: Develop efficient photocatalytic and electrochemical late-stage hydrogen isotope exchange strategies. Enhance sensitivity of tritium detection and miniaturize hydrogen isotope detection devices.
Methodology: We explore new synthetic routes for the introduction of hydrogen isotope markers to specific positions in organic molecules using advanced organic synthesis. Using functionalities in metal complexes, MOFs and organic molecules, we will create environments that enrich the local tritium concentration in adsorbed water, to enhance the sensitivity of scintillation measurements. We will further combine flow chemistry with scintillation and with fluorescence detection in order to monitor decaying tritium or its decay products in a lab-on-a-chip environment.
Compounds: organic molecules, non-covalent supramolecular networks, microstructured glass devices.
Associated courses: BC1, BC3, AC2, AC4.
Research projects: P5, P9, P10, P11, P12, P13, P14.
Research Projects
Our research programme encompasses 15 interdisciplinary research projects with strong synergy effects concerning the molecules and materials involved and the experimental and theoretical techniques applied.