Our research programme encompasses 15 interdisciplinary research projects with strong synergy effects concerning the molecules and materials involved and the experimental and theoretical techniques applied.
¹²³H’s Doctoral Research Projects
Each project is associated with one or more of the three research areas A-C and supervised by up to three principal investigators.
Hydrogen adsorption on isolated clusters and nanoparticles
Gas phase clusters serve as model systems for studying material properties under isolated conditions using the highly sensitive and selective toolkit of ion mass-spectrometry and laser spectroscopy. It is now possible to produce isolated clusters of complex composition, well-defined stoichiometry, mass, charge state and internal energy, and to characterize their structure, reactivity and dynamics isomer-specifically. In contrast, comparable methods for studying nanoparticle properties at the same level of detail are not yet available.
In project P1 we will characterize the role of nuclear quantum effects in the adsorption of dihydrogen isotopes by metal-containing clusters and microporous nanoparticles. The clusters will be characterized by infrared photodissociation spectroscopy and ion trap mass spectrometry. These results will then be used to understand the structure, kinetic and temperature-programmed desorption data obtained for the more complex nanoparticles. Ultimately, this project is aimed at improving the design concepts for dihydrogen isotopes separation materials.

![[Translate to English:] Single Nanoparticle Mass Spectrometer enlarge the image:](/fileadmin/_processed_/f/f/csm_p1_npinstrument_Reichhold_2a3dc61883.png)
In the Asmis Group, a longstanding experience exists with regard to combining mass spectrometric techniques with laser spectroscopy to characterize the cluster structure, reactivity and dynamics.[1,2] Our recent work has focused on the characterization of DHI and water adsorption by metal oxide clusters[3-5] in the context of molecular vs dissociative adsorption. In parallel, we have developed a novel method which allows characterizing the surface structure and adsorption behaviour of a single isolated NP by optically monitoring its mass variation non-destructively, as a function of temperature, background gas and interaction with electromagnetic radiation.[6,7] We are currently extending the technique to the IR spectral range.
References
- [1] H. Schwarz and K. R. Asmis, “Identification of Active Sites and Structural Characterization of Reactive Ionic Intermediates by Cryogenic Ion Trap Vibrational Spectroscopy”, Chem. Eur. J. 2019, 25, 2112-2126.
- N. Heine and K. R. Asmis, “Cryogenic ion trap vibrational spectroscopy of hydrogen-bonded clusters relevant to atmospheric chemistry”, Int. Rev. Phys. Chem. 2015, 34, 1-34. Corrigendium: 2016, 35, 507.
- [2] K. S. Lokare, B. Braun-Cula, C. Limberg, M. Jorewitz, J. T. Kelly, K. R. Asmis, S. Leach, C. Baldauf, I. Goikoetxea, J. Sauer, “Structure and Reactivity of Al−O(H)−Al Moieties in Siloxide Frameworks: Solution and Gas-Phase Model Studies”, Angew. Chem. Int. Ed. 2019, 58, 902-906.
- [3] X. Song, M. R. Fagiani, S. Debnath, M. Gao, S. Maeda, T. Taketsugu, S. Gewinner, W. Schöllkopf, K. R. Asmis, A. Lyalin, “Excess charge driven dissociative hydrogen adsorption on Ti2O4−”, Phys. Chem. Chem. Phys. 2017, 19, 23154-23161.
- [4] M. R. Fagiani, X. Song, S. Debnath, S. Gewinner, W. Schöllkopf, K. R. Asmis, F.A. Bischoff, F. Müller, J. Sauer, “Dissociative water adsorption by Al3O4+ in the gas phase”, J. Phys. Chem. Lett. 2017, 8, 1272-1277.
- [5] B. Hoffmann, T. K. Esser, B. Abel, K. R. Asmis, “Electronic Action Spectroscopy on Single Nanoparticles in the Gas Phase”, J. Phys. Chem. Lett. 2020, 11, 6051−6056.
- [6] T. K. Esser, B. Hoffmann, S. L. Anderson, K. R. Asmis, “A cryogenic single nanoparticle action spectrometer”, Rev. Sci. Instrum. 2019, 90, 125110 pp. 1-10.
Design of nanostructured materials for tritium capture
Tritium is among the most toxic isotopes and occurs as waste in heavy water reactors. It has been one of the major radioactive pollutants in the Fukishima incident. Having a half-life of less than 12 years, it can be treated within few human generations and does not require long-term deposition strategies. At the same time, tritium is one of the fuels needed for nuclear fusion, and it decays to the most precious nucleus, 3He, an essential material for ultra-cryogenic applications.
The separation of tritium from other hydrogen isotopes remains, however, a challenge. Today’s hydrogen isotope separation techniques are focused on the production of deuterium and have a rather low separation factor, which requires many separation cycles. For the separation of deuterium and tritium the separation factors are even lower, so the traditional processes become prohibitively inefficient, in particular given the radioactive nature of tritium in mind.
In the P2 project, we will computationally design structural motifs for hydrogen isotope separation, exploiting nuclear quantum effects, which are capable to capture tritium-containing isotopologues. These motifs will be realized in nanostructured materials such as metal-organic frameworks. We will determine the theoretically possible boundaries for the material’s performance for hydrogen isotope separation in terms of thermodynamics and kinetics.
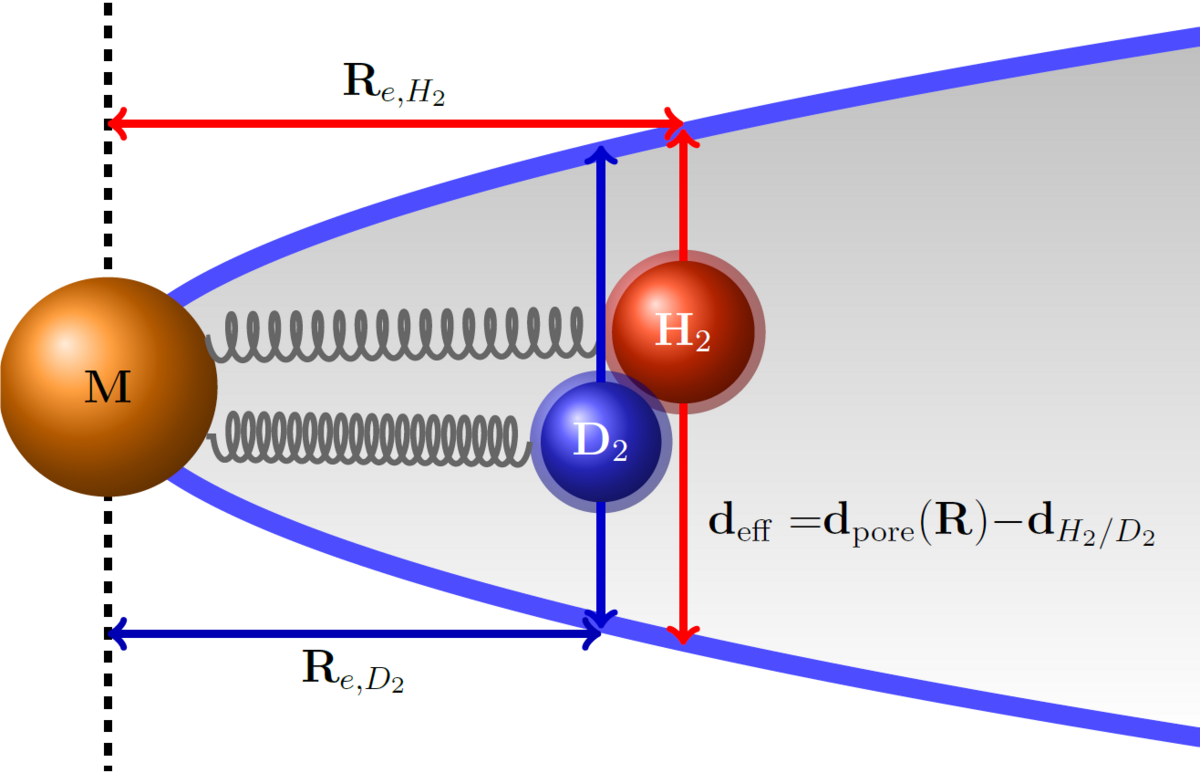
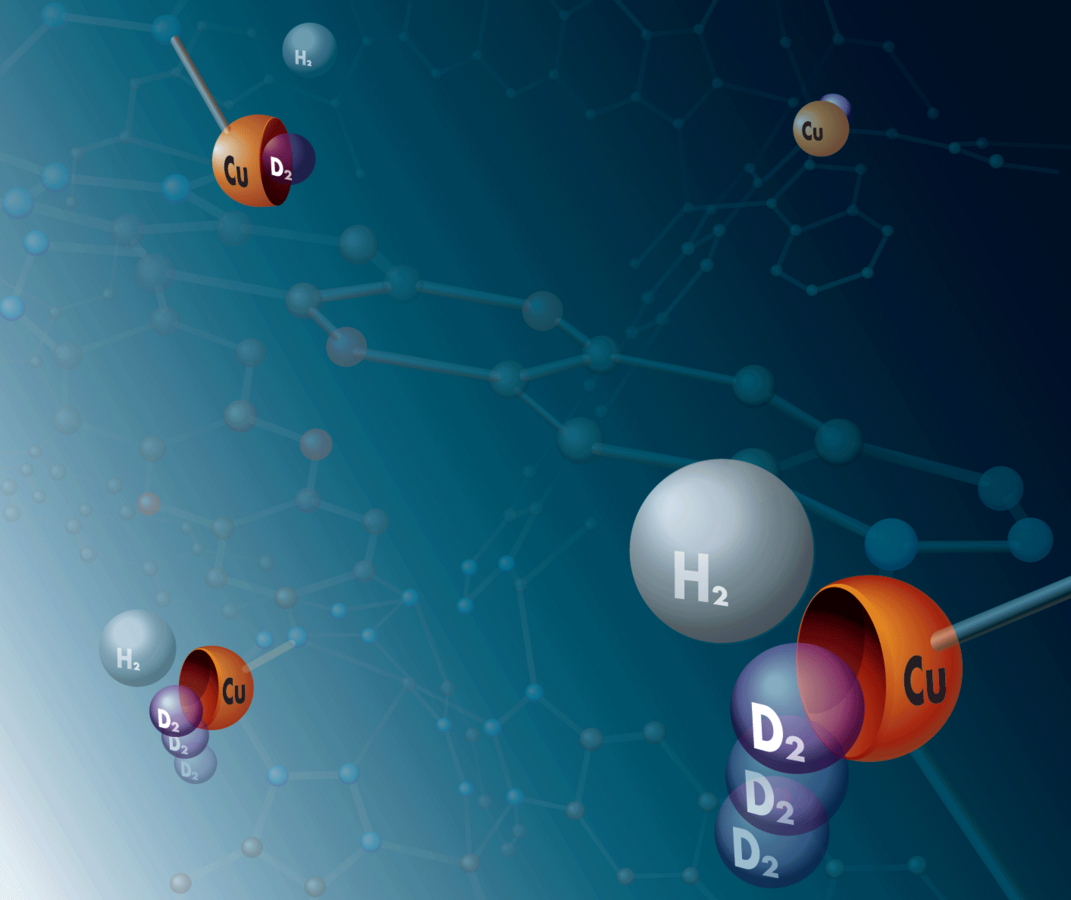
The Heine group is located at the Research Site Leipzig campus of HZDR and at TU Dresden with regular mutual visits of the subgroups. It offers excellent facilities for research, including an own compute cluster, and maintains an active national and international research network. For details on the group interests please visit the Heine group homepage.
References
- [1] I. Weinrauch, I. Savchenko, D. Denysenko, S. M. Souliou, H. Kim, M. Le Tacon, L. Daemen, Y. Cheng, A. Mavrandonakis, A. Ramirez-Cuesta, D. Volkmer, G. Schütz, M. Hirscher, T. Heine, “Capture of heavy hydrogen isotopes in a metal-organic framework with active Cu(I) sites”, Nat. Commun. 2017, 8, 14496.
Molecular framework materials with strongly attractive dihydrogen adsorption sites
Motivation and state of the art. MOFs with their high porosity and internal surface are suitable materials for separation of gases by adsorption. The motivation of this project, aimed at the development of MOF materials with strong binding sites for dihydrogen adsorption, is based on the observation of extraordinary high H2 adsorption enthalpy for CuI-MFU-4l, a MOF with low-coordinated CuI atoms in funnel-shaped pores. Due to nuclear quantum effects, the adsorption enthalpy is even higher for heavier dihydrogen isotopologues and allows H2/D2 separation by adsorption and desorption at temperatures above the boiling point of liquid nitrogen (77 K). Low coordinated CuI sites have also been detected as structural defects in HKUST-1 SURMOFs (Cu3(btc)2 deposited as thin layers). Such defects in CuII2(O2CR)4 paddle wheel units can be generated on purpose, e.g., in HKUST-1 by synthesis using a mixture of H3btc and isophthalic acid or 2,6-pyridine dicarboxylic acid instead of pure H3btc. This results in incomplete paddle wheel units CuICuII(O2CR)3 with reduced copper atoms (CuI) and unoccupied coordination sites.
Own work. The Krautscheid group has years of experience in synthesis and structural characterization of microporous MOFs. Typical linkers with anionic benzoate or isophthalate and neutral triazole groups provide various possibilities for derivatisation. Linker substitution, anion variation and mixed metal systems are used to modify structural details and to adjust adsorption properties.[1,2] E.g., [CuII(Mepy-trz-ia)] is among the porous materials with the highest hydrogen uptake at atmospheric pressure (3.1 wt-% at 77 K).[3] Inelastic neutron scattering (INS) studies at different H2 loadings have provided evidence for simultaneous occupation of several adsorption sites at the inner surface.[4] Recent investigations deal with structurally flexible MOFs and the observation of their gate opening behavior by in situ powder X-ray diffraction. By variation of the substituent R in triazole-isophthalate paddle wheel MOFs [Cu2(R-trz-ia)2] size and shape of the micropores, structural flexibility and gate opening behavior during gas adsorption can be adjusted.[2] Structural defects (missing Cu atoms) have been observed in various CuII MOFs with paddle wheel structures. Spectroscopic evidence for such defective Cu2(O2CR)4 units has been provided by EPR investigations.[5]
Aims and work plan. This project aims at the construction of MOFs that allow extremely strong interactions with adsorbed dihydrogen molecules which is the prerequisite for adsorption/desorption based DHI separation. The frameworks will be modified for high adsorption enthalpies exploiting the results of quantum chemical calculations, which suggest strongly attractive H2 adsorption sites by generating coordinatively unsaturated CuI sites in a funnel-shaped environment. Strategies to form such sites include syntheses
using a mixture of the regular and a modified “defective” protonated linker (please see figure below). Thus, the missing donor group is expected to provide the desired funnel-shaped cavity similar to that in CuI-MFU-4l leading to highly attractive hydrogen adsorption sites. Another approach considers the reduction of CuII to CuI in MOFs with CuII3(μ3-OH)(μ3-anion) SBUs (anion = sulfate, selenate, nitrate)[6] accompanied by elimination of the coordinating anion also generating unoccupied coordination sites.

Close collaborations will connect this MOF-synthesis project to theory and investigation of model systems. Theoretical investigations will predict metal/linker/defect linker systems that provide high H2 adsorption enthalpies and quantum effects for DHI separation. On the other hand, experimentally obtained (“defective”) MOFs will be modeled by DFT methods. Spectroscopic measurements will be performed on gas phase clusters as models for adsorption sites in MOFs. Thin films of tailored model compounds will be generated by the novel ion soft-landing technique. Hydrogen adsorption experiments will be conducted in collaboration with project partners. Local information about open metal sites and adsorption sites can be obtained by EPR and ENDOR methods, if paramagnetic ions are involved.
References
- [1] J. Bergmann, K. Stein, M. Kobalz, M. Handke, M. Lange, J. Moellmer, F. Heinke, O. Oeckler, R. Gläser, R. Staudt, H. Krautscheid, „A series of isomorphous metal-organic frameworks with rtl topology – Metal distribution and tunable sorption capacity via substitution of metal ion”, Micropor. Mesopor. Mater. 2015, 216, 56-63.
- [2] M. Kobalz, J. Lincke, K. Kobalz, O. Erhart, J. Bergmann, D. Lässig, M. Lange, J. Möllmer, R. Gläser, R. Staudt, H. Krautscheid, „Paddle wheel based triazolyl isophthalate MOFs: Impact of linker modification on crystal structure and gas sorption properties”, Inorg. Chem. 2016, 55, 3030-3039.
- [3] D. Lässig, J. Lincke, J. Möllmer, C. Reichenbach, A. Möller, R. Gläser, G. Kalies, K. A. Cychosz, M. Thommes, R. Staudt, H. Krautscheid, „A microporous copper metal-organic framework with high H2 and CO2 adsorption capacity at ambient pressure”, Angew. Chem Int. Ed. 2011, 50, 10344-10348.
- [4] O. Erhart, P. A. Georgiev, H. Krautscheid, „Desolvation process in the flexible metal-organic framework [Cu(Me-4py-trz-ia)], adsorption of dihydrogen and related structure response”, CrystEngComm 2019, 21, 6523-6535.
- [5] S. Friedländer, M. Simenas, M. Kobalz, P. Eckold, O. Ovchar, A. G. Belous, J. Banys, H. Krautscheid, A. Pöppl, “Single crystal electron paramagnetic resonance with dielectric resonators of mononuclear Cu2+ ions in a metal-organic framework containing Cu2 paddle wheel units”, J. Phys. Chem. C 2015, 119, 19171-19179.
- [6] J. Lincke, D. Lässig, M. Kobalz, J. Bergmann, M. Handke, J. Möllmer, M. Lange, C. Roth, A. Moeller, R. Staudt, H. Krautscheid, „An isomorphous series of cubic, copper-based triazolyl isophthalate MOFs: Linker substitution and adsorption properties”, Inorg. Chem. 2012, 51, 7579-7586.
Characterization of adsorbed dihydrogen isotopes in porous materials by hyperfine spectroscopy
Free metal ion centers in nanoporous materials (MOFs, zeolites) form effective adsorption sites for hydrogen isotopologues (DHI), which are essential for adsorption, separation and sieving processes. The direct spectroscopic characterization of the adsorbed DHI adsorbed at such metal sites appears to be still a challenge. In case of paramagnetic ions serving as adsorption sites pulse electron paramagnetic resonance (EPR) spectroscopy allows determination of the position and molecular states of the DHI species localized in close proximity of the metal ions.
In this project, we will characterize adsorption complexes of DHI with paramagnetic ions in MOF and zeolite materials by pulsed EPR methods to determine precisely the DHI positions within the host matrices from the measured 1H and 2D hyperfine couplings. Temperature dependent time domain electron spin echo envelope modulation spectroscopy (ESEEM) will provide supplementary information about desorption processes of the DHI on a local, microscopic scale.
The work of our group based at Felix-Bloch-Institute of Solid State Physics is devoted to the exploration of paramagnetic centers in solids and on solid surfaces. The investigation of the geometrical and electronic structure of adsorption complexes involving paramagnetic ions in MOFs and zeolites by cw and pulsed EPR spectroscopy has been a major research objective of our group in recent years. This work includes among other topics the characterization of D2 and HD adsorption complexes with Cu2+ ions in MOF HKUST-1 by pulsed electron nuclear double resonance (ENDOR) and hyperfine sublevel correlation spectroscopy (HYSCORE).
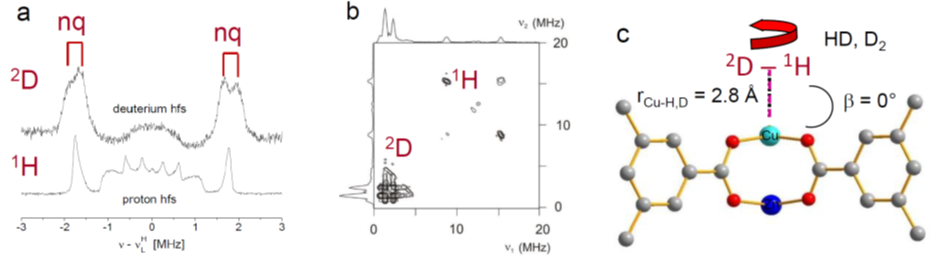
References
- [1] M. Mendt, M. Šimėnas, A. Pöppl: Electron paramagnetic resonance; in “The Chemistry of Metal-Organic Frameworks, Synthesis, Characterization, and Applications” Volume 2, Ed. S. Kaskel; Wiley-VCH Verlag GmbH & Co, Weinheim, (2016), ISBN 978-3-527-33874-0, pp. 629–565.
- [2] A. Palčić, P. C. Bruzzese, K. Pyra, M. Bertmer, K. Góra-Marek, D. Poppitz, A. Pöppl, R. Gläser, M. Jabłońska, “Nanosized Cu-SSZ-13 and its application in NH3-SCR”, Catalysts, 2020, 10, 506.-
- [3] B. Jee, M. Hartmann, A. Pöppl, “H2, D2 and HD adsorption upon the metal-organic framework [Cu2.97Zn0.03(btc)2]n studied by pulsed ENDOR and HYSCORE spectroscopy”, Mol. Phys. 2013, 111, 2950-2966.
- [4] M. Šimėnas, B. Jee, M. Hartmann, J. Banys, A. Pöppl, “Adsorption and desorption of HD on the metal−organic framework Cu2.97Zn0.03(Btc)2 studied by three-pulse ESEEM spectroscopy”, J. Phys. Chem. C, 2015, 119, 28530-28535.
- [5] Z. Fang, J. P. Dürholt, M. Kauer, W. Zhang, C. Lochenie, B. Jee, B. Albada, N. Metzler-Nolte, A. Pöppl, B. Weber, M. Muhler, Y. Wang, R. Schmid, R. A. Fischer, “Structural complexity in metal−organic frameworks: simultaneous modification of open metal sites and hierarchical porosity by systematic doping with defective linkers”, J. Am. Chem. Soc. 2014, 136, 9627-9636.
- [6] M. Mendt, F. Gutt, N. Kavoosi, V. Bon, I. Senkovska, S. Kaskel, A. Pöppl, “EPR insights into switchable and rigid derivatives of the metal−organic framework DUT-8(Ni) by NO adsorption”, J. Phys. Chem. C, 2016, 120, 14246–14259.
- [7] S. Friedländer, M. Šimėnas, M. Kobalz, P. Eckhold, O. Ovchar, A. F. Belous, J. Banys, H. Krautscheid, A. Pöppl, “Single crystal electron paramagnetic resonance with dielectric resonators of mononuclear Cu2+ ions in a metal-organic framework containing Cu2 Paddle Wheel Units”, J. Chem. Phys. C, 2015, 119, 19171-19179.
Novel materials for dihydrogen isotope separation generated by soft-landing of mass-selected molecular ions
Ion soft-landing is a method that allows the deposition of mass-selected ions on surfaces under vacuum. The recent development of new electrospray ionization (ESI) sources has significantly increased available ion fluxes and allows the generation of thin material layers.[1,2] This opens new possibilities of harnessing gaseous molecular ions for the generation of functional material layers. MOFs and zeolites have been introduced as “bulk” materials which can carry under-coordinated (cationic) metal centers for dihydrogen isotope (DHI) separation. We are going to generate new model systems for DHI separation-materials by using ion soft-landing.
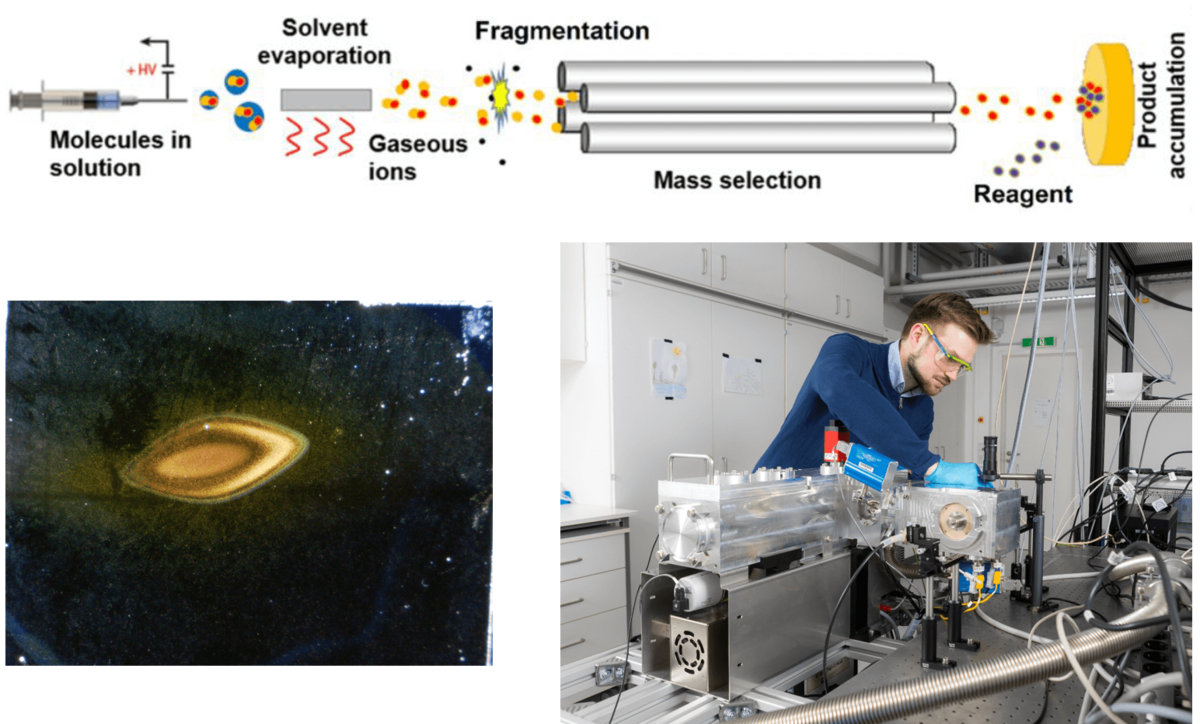
Work plan: (i) Metal cations and linkers used for the preparation of DHI-separating MOFs (see project P3) will be soft landed separately. We are going to characterize this new material to understand if and how MOF structures with favorable DHI separation properties may be designed by this method. (ii) We are going to modify thin layers of zeolites with soft-landed ions to increase their DHI-separation ability (in cooperation with project P4). (iii) We explore the preparation of alternative materials containing under-coordinated metal cations as DHI binding centers. Free coordination sites in metal organic compounds will be generated by fragmentation reactions in the gas phase. We will search for methods which allow the stabilization of these fragment ions after their landing on surfaces. Information on the most promising gaseous cationic candidates will be provided by projects P1 and P2.
The group: We are a junior research group including doctoral students and postdocs with chemistry and physics background. Our work is pioneering in using molecular fragment ions for the generation of new materials on surfaces.[2,3] We provide intensive support and knowledge transfer in the fields of instrumentation development, mass spectrometry, molecular analysis and material characterization, reactivity of gaseous ions and computational investigations. For details on our projects please visit the group page of the NFG Warneke.
References
- [1] J. Laskin, G. E. Johnson, J. Warneke, V. Prabhakaran, “From isolated ions to multilayer functional materials using ion soft-landing” Angew. Chem. Int. Ed. 2018, 57, 16270-16284.
- [2] J. Warneke, M. E. McBriarty, S. L. Riechers, S. China, M. H. Engelhard, E. Aprà, R. P. Young, N. M. Washton, C. Jenne, G. E. Johnson, J. Laskin, “Self-organizing layers from complex molecular anions” Nat. Commun. 2018, 9, 1889.
- [3] J. Warneke, M. Mayer, M. Rohdenburg, X. Ma, J.K.Y. Liu, M. Grellmann, S. Debnath, V. A. Azov, E. Aprà, R. P. Young, C. Jenne, G. E. Johnson, H. I. Kenttämaa, K. R. Asmis, J. Laskin “Direct functionalization of C-H bonds by electrophilic anions” Proc. Natl. Acad. Sci. U.S.A. 2020, 117, 23374-23379.
Investigation of electrochemically and photon driven hydrogen isotope penetration through thin membranes
Motivation and state of the art. Graphene and hexagonal boron nitride monolayers are impermeable to thermal atoms and molecules, but permeable to hydrogen isotope nuclei. The origin of the reported isotope effect is still not completely understood yet. Because graphene monolayers are surprisingly transparent to protons (deuterons), there was growing interest to use it as a proton-conducting membrane in fuel cells. Surprisingly, proton transport through Pt-nanoparticle-decorated graphene can be significantly enhanced by light, with a gain of about 10000 protons per photon. However, a full understanding of the effect is also still missing here.
Own work. The Abel group has a long-term experience in microfluidics, microfluidic fabrication, molecular electrochemistry,[1-8] various techniques of high-resolution mass spectrometry, many spectroscopic technologies, as well as mechanistic investigations based upon quantum theory. Recently, a number of publications have been focusing on graphene and graphene oxide[3,4,9,10] as well as multilayer materials separating/protecting catalyst and electrolyte in fuel cells with electron and proton conducting properties. The Abel group has a large experience to realize microfluidic electrochemical cells, such as employed in this project. A special research focus over the past 15 years is in the sensitive detection of traces in gases and fluids. The project and the RTG will largely benefit from these combined experiences and expertise, and from the experience of synthesizing thin films and the decoration of thin films such as graphene with atomic layer deposition (ALD). Most of the experiments in the Abel group recently have been accompanied by theoretical modelling,[5,7] allowing for detailed mechanistic insights. [9,10]
Aims and work plan. The central objective of the project P7 is to understand and quantify proton/deuteron transport, as well as its enhancement by photons through thin single- or multilayer membranes and to derive a mechanistic understanding of the effect. The proton/deuterium transfer through membranes and subsequent transformation to H2/D2 will be detected in operando employing ultra-sensitive mass spectrometry. This will allow us to understand the potential of the approach in terms of hydrogen isotope separation technologies. Furthermore, a compact solution may enable us to couple the technology to other analytical, enrichment and storage modules.
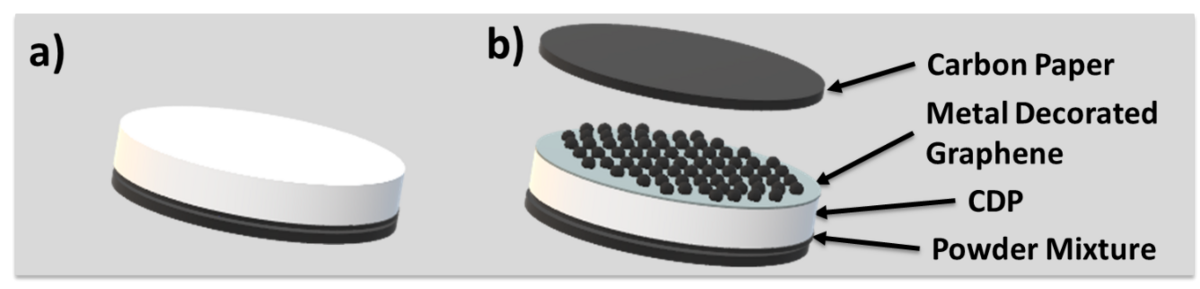
First, we employ a Geim-type cell with the goal of confirming and extending the reported experiments and results, as well as to derive a mechanistic understanding of the effect (together with P2 and P8). Second, we aim to investigate the giant photo effect reported very recently. A mechanistic understanding is also missing here and in the focus of the work in P7.
In contrast to earlier work, we have very recently found (unpublished work) that proton (and possibly D+ and T+) diffusion and penetration can indeed proceed even through membranes of multilayer graphene oxide. Therefore, we plan to employ a novel all solid-state (solid acid) fuel cell, enabling us to measure an isotope effect of hydrogen isotope nuclei penetrating through multilayer graphene oxide membranes (electrochemical setup). Finally, we will employ the fuel cell setup for tritium in a dedicated setup at HZDR/FSL (with P12).
References
- [1] O. Naumov, S. Naumov, R. Flyunt, B. Abel, A. Varga, “Fast degradation for high activity: Oxygen- and nitrogen-functionalized carbon nanotubes in solid-acid fuel-cell electrodes”, ChemSusChem 2016, 9, 1-10.
- [2] P. S. With, U. Helmstedt, S. Naumov, A. Sobottka, U. Decker, A. Prager, R. Heller, B. Abel, L. Prager, “Low temperature photochemical conversion of organometallic precursor layers to titanium(IV) oxide thin films”, Chem. Mater. 2016, 28, 7715-7724.
- [3] A. Kahnt, R. Flyunt, C. Laube, W. Knolle, S. Eigler, R. Hermann, S. Naumov, B. Abel, “How fast is the reaction of hydrated electrons with graphene oxide in aqueous dispersions?”, Nanoscale 2015, 7, 19432-19437.
- [4] R. Flyunt, W. Knolle, A. Kahnt, A. Prager, A. Lotnyk, J. Malig, D. Guldi, B. Abel, “Mechanistic aspects of the radiation-chemical reduction of graphene oxide to graphene-like materials”, Int. J. Radiat. Biol. 2014, 90, 486-494.
- [5] O. Naumov, S. Naumov, B. Abel, A. Varga, “The stability limits of highly active nitrogen doped carbon nanotubes ORR catalysts: A mechanistic study of degradation reactions”, Nanoscale 2018, 10, 6724-6733.
- [6] F. P. Lohmann-Richters, B. Abel, A. Varga, “In situ determination of the electrochemically active platinum surface area: Key to improvement of solid acid fuel cells”, J. Mater. Chem. A 2018, 6, 2700-2707.
- [7] O. Naumov, F. P. Lohmann, B. Abel, A. Varga, “Carbon nanotubes as a solid acid fuel cell cathode material: Insights into in operando functional stability”, ChemElectroChem 2017, 4, 1306-1313.
- [8] F. P. Lohmann, P. S. C. Schulze, M. Wagner, O. Naumov, A. Lotnyk, B. Abel, A. Varga, “The next generation solid acid fuel cell electrodes: stable, high performance with minimized catalyst loading”, J. Mater. Chem. A 2017, 5, 15021-15025.
- [9] R. Flyunt, W. Knolle, A. Kahnt, C. E. Halbig, A. Lotnyk, T. Häupl, A. Prager, S. Eigler, B. Abel, “High quality reduced graphene oxide flakes by fast kinetically controlled and clean indirect UV-induced radical reduction”, Nanoscale 2016, 8, 7572-7579.
- [10] A. Kahnt, R. Flyunt, S. Naumov, W. Knolle, S. Eigler, R. Hermann, B. Abel, “Shedding light on the soft and efficient free radical induced reduction of graphene oxide: hidden mechanisms and energetics”, RSC. Adv. 2016, 6, 68835-68845.
Fundamental understanding of hydrogen diffusion and sieving (B)
A few years ago, high selectivity for proton over deuteron diffusion through membranes of two-dimensional crystals, such as graphene, have been reported experimentally. Soon after, the same group has shown that hydrogen isotope separation is possible in the interstitial space between the layers of 2D materials. Both experiments raised important questions to be answered: is graphene permeable to protons? Which particles are transported (ions or atoms) between layers of 2D materials? Recently, we have addressed and answered both above mentioned questions.
In [1], we have shown that, indeed, perfect graphene without any atomic defects is impermeable to protons. However, a small concentration of so-called Stone-Wales (55-77 SW) defects lowers significantly the energy barrier for hydron penetration and explains well the H+/D+ selectivity ratio. Here, we have taken into account nuclear quantum effects (NQEs), namely tunnelling, using the WKB (Wentzel–Kramers–Brillouin) approach and in-house written code for transmission probabilities. The code works also for other isotopes. In [2], we have simulated atomic and ionic transport of hydrogen species between 2D layers, supporting the fact that hBN and MoS2 are favourable materials for this purpose, while graphite showed suppressed transport. The transported species are atoms, which is unfortunate, because only small concertation can be handled to avoid recombination to H2 molecules in between layers.
Here, we will study diffusion of protons and other H isotopes through other 2D membranes, such as transition metal dichalcogenides (TMDCs), twisted layers of graphene or hBN, or their heterostructures (see Figure 1). We will estimate possible tunnelling of the species, as well as, energy barrier lowering due to defect formation. Later, we will extend our study to 2D noble-metal dichalcogenides (NMDCs), based on Pt or Pd,[3] as well as to the 2D molecular framework membranes, i.e., 2D COFs, as they offer regular pore size tuning, which could be exploited in kinetic quantum sieving. Moreover, we will support experimental investigations on the diffusion processes through the metal-decorated membranes.

The Kuc group is located at the Research Site Leipzig campus of HZDR. It offers excellent facilities for research, including an own compute cluster, and maintains an active national and international research network. For subjects of the group, please visit: https://www.researchgate.net/profile/Agnieszka-Kuc
References
- [1] Y. An, A. F. Oliveira, T. Brumme, A. Kuc, T. Heine, “Stone-Wales defects cause high proton permeability and isotope selectivity of single-layer graphene“, Adv. Mater. 2020, 32, 2002442.
- [2] Y. An, A. Kuc, P. Petkov, M. Lozada-Hidalgo, T. Heine, “On the chemistry and diffusion of hydrogen in the interstitial space of layered crystals h-BN, MoS2, and graphite“, Small 2019, 15, 1901722.
- [3] R. Kempt, A. Kuc, T. Heine, “Two-dimensional noble-metal chalcogenides and phosphochalcogenides“, Angew. Chem. Int. Ed. 2020, 59, 9242–9254.
Photocatalytic deuteration & tritiation in batch and flow using water isotopologues
Labelled compounds are important tools for analytics, standardization, and mechanistic studies, including the tracing of bioactive compounds and are typically employed for the optimization of drug candidates within ADME studies. Late-stage functionalization approaches, including hydrogen isotope exchange (HIE) can strongly contribute to the improved availability of D/T-labelled compounds. Visible light photocatalysis has emerged as a powerful tool for late-stage transformations; several promising examples for isotopic labelling have been demonstrated recently.[1] Selective catalytic, visible light photoredox protocols for nH labelling of various structural motifs via late-stage deuterations and tritiations using isotopically labelled water as the source for hydrogen isotopologues will be pursued.
Building on the intrinsic selectivity of photoredox catalytic reactions, we aim to develop oxidative and reductive approaches for the isotopic labelling of compounds with diverse, structural abundant motifs using multicatalytic strategies. Experiments will first be conducted for deuterium incorporation, but will later be extended to T-labelling (in cooperation with P12). Advantages of flow approaches for improved performance and direct analysis will be studied in cooperation with P11. Our synthetic studies will benefit from an improved understanding of isotopic effects in H-bonding networks of different isotopologues together with P13 and P14.
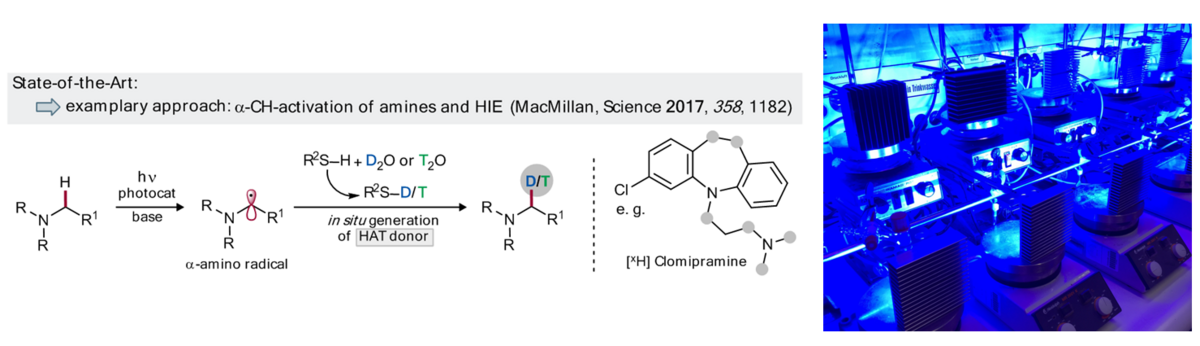
For this position, we are looking for an open-minded, highly motivated chemist with a solid background in preparative organic chemistry and with a strong interest in photocatalysis, but also in synthesis and mechanistic studies (together with our RTG collaboration partners). Prior experience in photochemistry and/or –catalysis is beneficial.
The Zeitler group has a long-term experience in the area of photoredox catalysis, in particular in the investigation of different multicatalytic photoredox approaches, including combinations with hydrogen atom transfer (HAT), hydrogen-bond activation,[2] enamine[3] or Lewis acid catalysis.[4] We have developed novel methodology to address the challenging fission of strong bonds,[5-8] as well as a toolbox of new purely organic photocatalysts.[3, 8] Our mechanistic studies also include photochemical flow approaches.[9]
References
- [1] H. Li, M. Shabbir, W. Li, A. Lei, ”Recent Advances in Deuteration Reactions“, Chin. J. Chem. 2024, 42, 1145-1156.
[2] M. Neumann, K. Zeitler, “A cooperative hydrogen-bond-promoted organophotoredox catalysis strategy for highly diastereoselective, reductive enone cyclization“, Chem. Eur. J. 2013, 19, 6950-6955.
[3] M. Neumann, S. Füldner, B. König, K. Zeitler, “Metal-free, cooperative asymmetric organophotoredox catalysis with visible light”, Angew. Chem. Int. Ed. 2011, 50, 951-954.
[4] E. Speckmeier, P. J. W. Fuchs, K. Zeitler, “A synergistic LUMO lowering strategy using Lewis acid catalysis in water to enable photoredox catalytic, functionalizing C‑C cross-coupling of styrenes“, Chem. Sci. 2018, 9, 7096-7103.
[5] E. Speckmeier, C. Padié, K. Zeitler, “Visible light mediated reductive cleavage of C–O bonds accessing α-substituted aryl ketones”, Org. Lett. 2015, 17, 4818-4821.
[6] E. Speckmeier, K. Zeitler, “Desyl and phenacyl as versatile, photocatalytically cleavable protecting groups – A classic approach in a different (visible) light”, ACS Catal. 2017, 7, 6821-6826.
[7] E. Speckmeier, M. Klimkait, K. Zeitler, “Unlocking the potential of phenacyl protecting groups: CO2-based formation and photocatalytic release of caged amines”, J. Org. Chem. 2018, 83, 3738-3745.
[8] E. Speckmeier, T. G. Fischer, K. Zeitler, “A toolbox approach to construct broadly applicable metal-free catalysts for photoredox chemistry – Deliberate tuning of redox potentials and importance of halogens in donor-acceptor cyanoarenes“, J. Am. Chem. Soc. 2018, 140, 15353-15365.
[9] M. Neumann, K. Zeitler, “Application of microflow conditions to visible light photoredox catalysis“, Org. Lett. 2012, 14, 2658-2661.
Electrochemical xH-labelling in microheterogeneously structured solutions
Project: Late-stage hydrogen isotope exchange (HIE) is a versatile and mild alternative to classical, often laborious and expensive labelling strategies, which often suffer from low functional group tolerance and random incorporation of multiple D/T atoms. Methods aiming at a generally applicable and efficient late-stage conversion of one specific C-H into a C-D/T bond are highly desirable but at the same time highly challenging. Electrochemistry is a versatile platform for selective Csp2-H functionalization as recently demonstrated for oxidative C-C coupling. Fluorinated alcohols, such as HFIP, are key for the successful oxidation of aryls, due to their ability to form cooperative H-bonding networks together with Lewis basic molecules and thus help to achieve selective bond activation and radical(cation) stabilization. Based on these beneficial cooperative effects, electrochemical HIE using D2O as labelling reagent will be established.
Supramolecular assemblies consisting of F-alcohols and xH2O will be generated and utilized as tools for selective HIE in aromatic compounds with our synthetic efforts focusing on sophisticated, yet electrochemically less explored, heterocyclic substrates as such structural entities are the most ubiquitous structural motifs in bioactive compounds. All experiments will first be conducted with the easier to handle D2O before the T-labelled analogs (collaboration P12) will be applied. Our preparative efforts will thus be accompanied by characterization of the isotopologues-dependent H-bonding networks together with P13 and P14.

Our lab: The Gulder group has experience in biomimetic catalysis with focus on halogenations. Besides developing selective C-X bond methods, we have significantly contributed to the understanding of supramolecular F-alcohol-Lewis base H-bonding networks and their use as activating and directing elements to trigger novel reactivities and selectivities. Lately, the Gulder group has contributed to the field of electrochemical fluorinations, a currently hot topic in organic chemistry as it offers new, environmentally benign synthetic pathways difficult to access by classic organic synthesis.has experience in biomimetic catalysis with focus on halogenations. Besides developing selective C-X bond methods, we have significantly contributed to the understanding of supramolecular F-alcohol-Lewis base H-bonding networks and their use as activating and directing elements to trigger novel reactivities and selectivities. Lately, the Gulder group has contributed to the field of electrochemical fluorinations, a currently hot topic in organic chemistry as it offers new, environmentally benign synthetic pathways difficult to access by classic organic synthesis.
References
- [1] A. Andries-Ulmer, C. Brunner, J. Rehbein, T. Gulder, “Fluorine as a traceless directing group for the regiodivergent synthesis of indoles and tryptophans”, J. Am. Chem. Soc. 2018, 140, 13034-13041.
- [2] S. V. Kohlhepp, T. Gulder, ”Hypervalent iodine(III) fluorinations of alkenes and diazo compounds: New opportunities in fluorination chemistry”, Chem. Soc. Rev. 2016, 45, 6270-6288.
- [3] A. Ulmer, C. Brunner, A. M. Arnold, A. Poethig, T. Gulder, “A fluorination/aryl migration/cyclization cascade for the metal-free synthesis of fluoro-benzoxazepines”, Chem. Eur. J. 2016, 22, 3660-3664.
- [4] A. M. Arnold, A. Poethig, M. Drees, T. Gulder, “NXS, morpholine, and HFIP: The ideal combination for biomimetic haliranium-induced polyene cyclizations”, J. Am. Chem. Soc. 2018, 140, 4344-4353.
A lab-on-a-chip platform for detection of tritium species at microscale
Tritium is a preferred radiolabel and widely applied in drug metabolism studies. Tritium-labelled compounds can be detected at high sensitivity applying liquid scintillation counting (LSC), which is, in principle, also possible in continuous flow. The standard on-line radioactivity detection cells are, however, not compatible with micro- or even nano-flow rates of modern HPLC-systems. Miniaturisation of in-flow scintillation assays addresses this challenge and would also lead to a drastic reduction of required sample amount and radioactive waste. This is envisioned by the development of integrated chip laboratories seamlessly combining microflow reactors, HPLC-columns, and scintillation detection. Such integrated microsystems can significantly improve analytical performance, safety, and sustainability.
In project P11 we will develop enabling technologies for sensitive LSC detection of tritium species at the microscale. The approach should facilitate seamless system integration with upstream processes like HPLC and continuous flow reactions. The novel approach for liquid scintillation counting on-chip shall possess the following specifics: (1) Minimal reagent consumption such as of radioactive analytes and scintillation liquids; (2) Minimising exposure and radioactive waste; (3) On-line radio-monitoring in continuous microflow; (4) Seamless coupling with upstream HPLC-separation; (5) Hyphenation to microflow reactor systems.
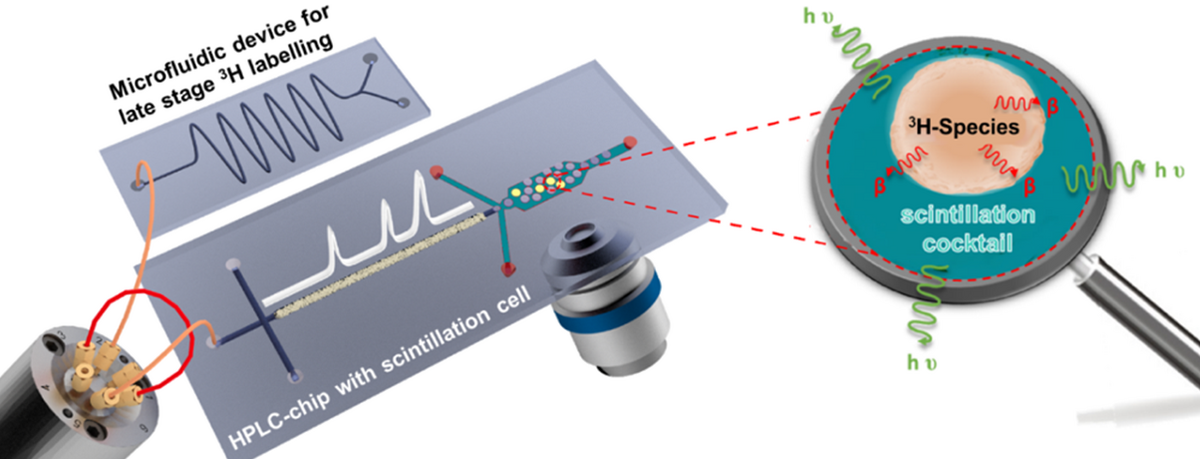
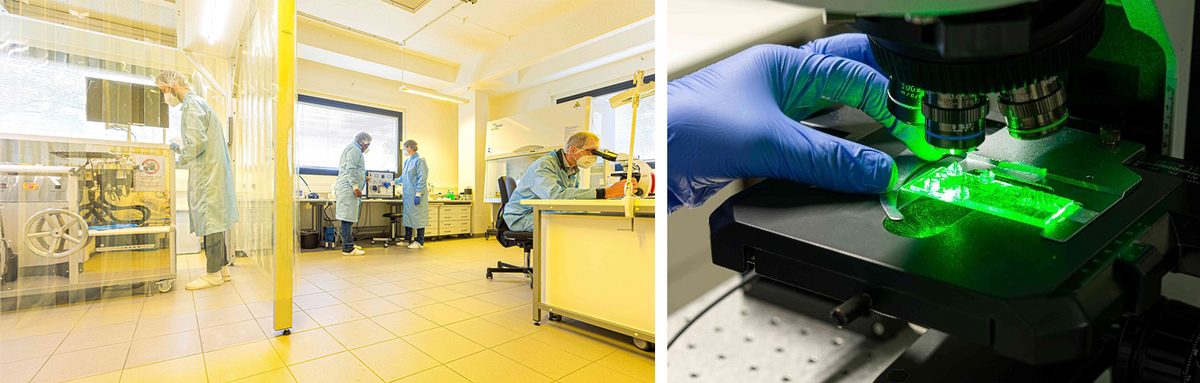
These lab-on-a-chip systems are developed in-house and manufactured in clean rooms (left picture) using state-of-the-art micromachining technologies. The chips produced are examined using microspectroscopic techniques such as fluorescence and Raman microspectroscopy. They will also be combined with chip-HPLC and mass spectrometry as part of the project. A wide range of instruments, such as various laser microscopy devices and mass spectrometers, are available for this purpose. Moreover, also new approaches for microscintillation detection will be investigated in the context of the project.
In the Belder group, we are specialised in developing integrated microdevices combining chemical conversion, separation, and detection on microfluidic chips [1-7]. To realise such systems, the group can resort to a long-standing expertise in the development and prototyping of lab-on-chip devices and coupling of microfluidic chips with spectroscopic techniques.
References
- [1] K. M. Krone, R. Warias, C. Ritter, A. Li, C. G. Acevedo-Rocha, M. T. Reetz, D. Belder, “Analysis of Enantioselective Biotransformations Using a Few Hundred Cells on an Integrated Microfluidic Chip”, J. Am. Chem. Soc. 2016, 138, 2102-2105.
- [2] R. Gerhardt, A. J. Peretzki, S. K. Piendl, D. Belder, “Seamless combination of high pressure chip-HPLC and droplet microfluidics on an integrated microfluidic glass chip”, Anal. Chem. 2017, 89, 13030-13037.
- [3] T. A. Meier, R. J. Beulig, E. Klinge, M. Fuss, S. Ohla, D. Belder, “On-chip monitoring of chemical syntheses in micro-droplets via surface-enhanced Raman spectroscopy”, Chem. Commun. 2015, 51, 8588-8591.
- [4] K. Piendl, T. Schönfelder, M. Polack, L. Weigelt, T. van der Zwaag, T. Teutenberg, E. Beckert, D. Belder, “Integration of Segmented Microflow Chemistry and Online HPLC/MS Analysis on a Microfluidic Chip System Enabling Enantioselective Analyses at the Nanoliter Scale”, Lab Chip 2021, DOI: 10.1039/D1LC00078K
- [5] A. J. Peretzki, S. Schmidt, E. Flachowsky, A. Das, R. F. Gerhardt, D. Belder, “How electrospray potentials can disrupt droplet microfluidics and how to prevent this”, Lab Chip, 2020, 20, 4456-4465.
- [6] J. J. Heiland, R. Warias, C. Lotter, P. Fuchs, L. Mauritz, K. Zeitler, S. Ohla, D. Belder, “On-chip integration of organic synthesis and HPLC/MS analysis for monitoring stereoselective transformations at the micro-scale”, Lab Chip 2017, 17, 76-81.
- [7] R. Warias, D. Ragno, A. Massi, D. Belder, “A visible‐light‐powered polymerization method for the immobilization of enantioselective organocatalysts into microreactors“ Chem. Eur. J. 2020, 26, 13152-13156.
Benchmarking microfluidic T detection methods; basic separation studies with hydrogen isotope mixtures including T
The heaviest hydrogen isotope, tritium (3T), is also the heaviest challenge from an experimental and analytical point of view. In its elemental form, it is a radioactive gas with an activity of 1011 Bq (decays per second) in just one milliliter, seeking all opportunities to escape from containments. Safe handling requires appropriate skills and safety measures. On the other hand, the same features make it possible to detect this isotope at quantities that are (by far!) not reached by conventional analytical methods.
Experimental work in project P12 is closely linked to the topics of P7 (membrane separation) and P11 (T detection). Including T2 in separation studies shall provide a comprehensive experimental basis to understand the selective performance of new 2D membrane materials and to validate theoretical predictions about isotope separation efficiencies made in P2 and P8. The method of Liquid Scintillation Counting (LSC) is used for detection of T2 after oxidation, as well as for benchmarking microfluidic lab-on-a-chip techniques developed in P11.
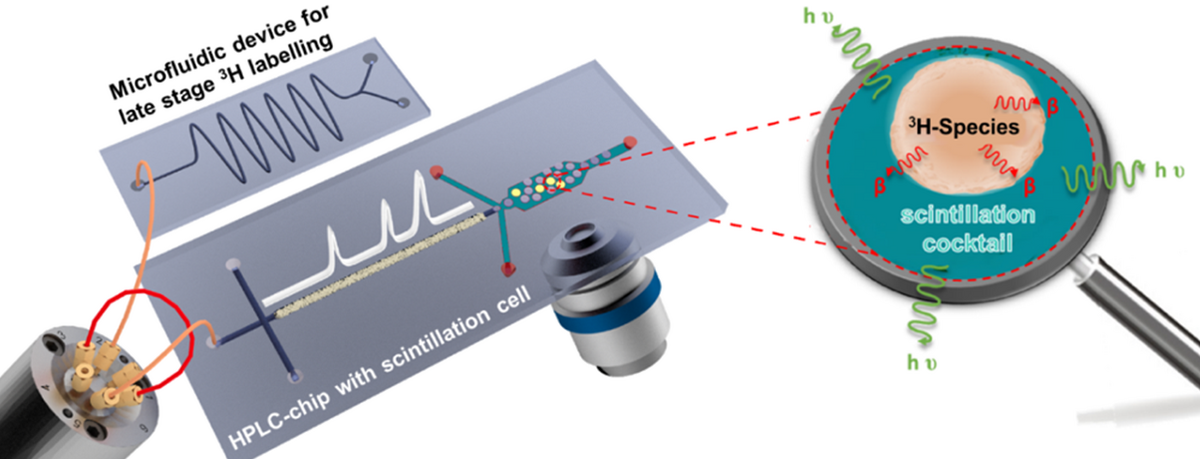
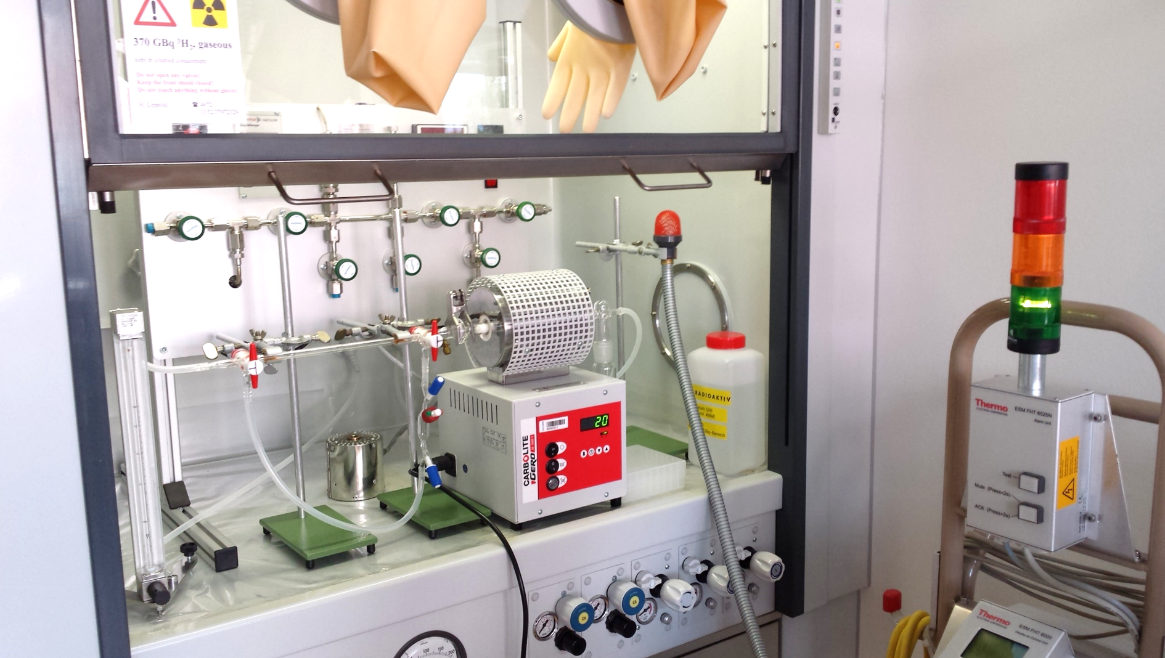
Supervised by experienced scientists, PhD students can take advantage of well-equipped radiochemical lab facilities located at the Research Site Leipzig of HZDR (Helmholtz-Zentrum Dresden-Rossendorf). The Reactive Transport group focuses on quantitative and predictive concepts with emphasis on fluid-solid interactions.[2, 3] Long-standing experience exists in the development and application of radioactive tracers including cyclotron-generated nuclides and low-energy beta emitters such as 3T.[4]
Doctoral position available at HZDR from 1 October 2024
For this position, we are looking for a chemist or physicist with a background in analytics, combined with the skills required in P7 or P11. Having established a reliable analytical routine by optimizing the conditions of reaction, transfer and trapping, systematic investigations of separation efficiencies will be possible, e.g., as a function of defect density in modified 2D membranes.
Learn more about this position at our ¹²³H Recruiting Symposium.
Apply for this position at HZDR
References
- [1] W. T. Shmayda, P. Mayer, “Uranium beds for temporary tritium storage”, J. Less Common Met. 1984, 104, 239-250.
- [2] C. Fischer, A. Lüttge, “Beyond the conventional understanding of water–rock reactivity”, Earth Planet. Sci. Lett. 2017, 457, 100-105.
- [3] C. Fischer, A. Lüttge, “Pulsating dissolution of crystalline matter”, PNAS 2018, 115 (5), 897-902
- [4] H. Lippold, J. Lippmann-Pipke, “New insights into the dynamics of adsorption equilibria of humic matter as revealed by radiotracer studies”, Geochim. Cosmochim. Acta 2014, 133, 362-371.
Isotopic fractionation in hydrogen-bonded clusters
The exploitation of nuclear quantum effects allows for isotopic fractionation, i.e., enrichment of one isotope (or isotopologue) relative to another. For hydrogen isotopes, these effects can be amplified in hydrogen-bonded networks. Prominent examples are the standard melting point of water, which is ~3.8 K higher in D2O than in H2O, the substantially higher mobility of protons vs. deuterons in aqueous solution (1.4:1.0) and, even more impressive, through single-layer graphene (14:1). However, predicting and modelling these effects is far from trivial.
Microhydrated gas-phase clusters serve as computationally tractable model systems for studying hydrogen-bond networks under isolated conditions using the highly sensitive and selective toolkit of gas-phase ion spectroscopy. They are amenable to higher-level calculations and thus prove useful for testing and benchmarking computational approaches. In project P13, we will study the influence of hydrogen-bonded networks on the selective binding of water isotopologues using clusters that mimic the binding environments of interest to the 1,2,3H.
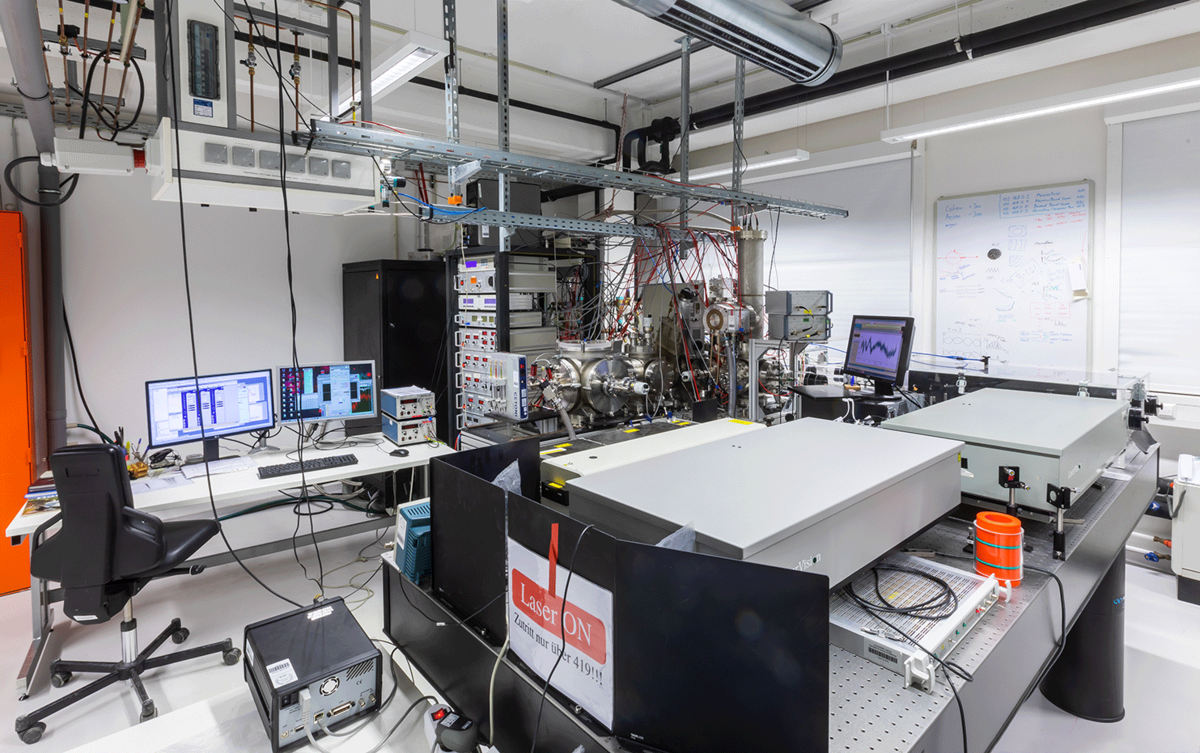
The Asmis group has pioneered the use of cryogenic ion vibrational spectroscopy (CIVS) to characterize microhydrated clusters in the gas phase.[1,2] CIVS has emerged as one of the most generally applicable and, at the same time, highly specific techniques to characterize the intrinsic cluster properties. It is now typically possible to produce microhydrated clusters of complex composition and probe them isomer-specifically. Our recent work has focused on the role of nuclear quantum effects in protonated water clusters[3,4] and the characterization of water adsorption by metal oxide clusters.[5,6]
References
- [1] H. Schwarz and K. R. Asmis, “Identification of active sites and structural characterization of reactive ionic intermediates by cryogenic ion trap vibrational spectroscopy”, Chem. Eur. J. 2019, 25, 2112-2126.
- [2] N. Heine and K. R. Asmis, “Cryogenic ion trap vibrational spectroscopy of hydrogen-bonded clusters relevant to atmospheric chemistry”, Int. Rev. Phys. Chem. 2015, 34, 1-34.
- [3] M. R. Fagiani, H. Knorke, T. Esser, N. Heine, C. T. Wolke, S. Gewinner, W. Schöllkopf, M.-P. Gaigeot, R. Spezia, M. A. Johnson, K. R. Asmis, “Gas phase vibrational spectroscopy of the protonated water pentamer: The role of isomers and nuclear quantum effects”, Phys. Chem. Chem. Phys. 2016, 18, 26743-26754.
- [4] C. T. Wolke, J. A. Fournier, L. C. Dzugan, M. R. Fagiani, T. T. Odbadrakh, H. Knorke, K. D. Jordan, A. B. McCoy, K. R. Asmis, M.A. Johnson, “Spectroscopic snapshots of the proton transfer mechanism in water”, Science 2016, 354, 1131-1135.
- [5] K. S. Lokare, B. Braun-Cula, C. Limberg, M. Jorewitz, J. T. Kelly, K. R. Asmis, S. Leach, C. Baldauf, I. Goikoetxea, J. Sauer, “Structure and reactivity of Al–O(H)–Al moieties in siloxide frameworks – solution and gas phase model studies”, Angew. Chem. Int. Ed. 2019, 58, 902-906.
- [6] M. R. Fagiani, X. Song, S. Debnath, S. Gewinner, W. Schöllkopf, K. R. Asmis, F.A. Bischoff, F. Müller, J. Sauer, “Dissociative water adsorption by Al3O4+ in the gas phase”, J. Phys. Chem. Lett. 2017, 8, 1272-1277.
Deep learning nuclear quantum effects for accelerated molecular dynamics
Isotope effects on chemical bonding have been heavily investigated for hydrogen-bonded systems, mainly for H2O/D2O. Competing quantum effects have been found, which either strengthen or weaken the hydrogen bond. The magnitude of the NQE strongly depends on bond length; thus, both X-H/D/T and X-H/D/T--Y distances play a decisive role. Much less studied are isotope effects on chemical bonding beyond the examples of H2O and D2O.
Now, the aim of this project is to develop methods to capture isotope effects on atomic and electronic structure of molecules beyond the example of water including all systems treated in the RTG ranging from organic molecules to extended surfaces.
The majority of computational studies of large molecular systems and surface chemistry is based on density functional theory (DFT). Modern DFT approaches will thus be applied to reach the project’s goal benchmarked by high-level wavefunction based methods including “gold standard” methods like CCSD(T) in recently developed efficient formulations. We will extend the methodology used to characterize chemical bonding to capture isotope effects. This will lead to progress in the theoretical understanding of isotope effects and provide connections to the other theoretical and the experimental projects in the RTG.
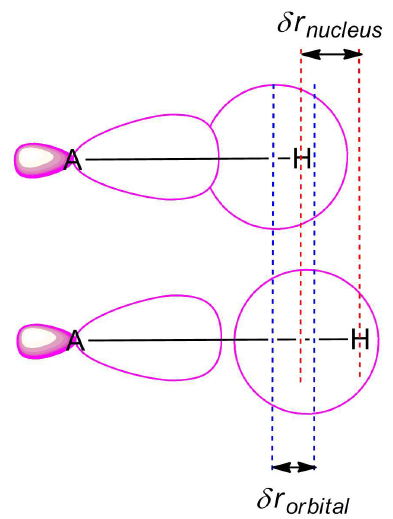
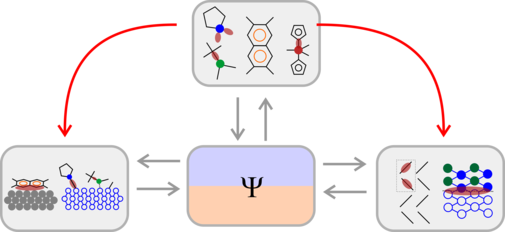
Analysis of chemical bonding in molecular and extended systems is a major research focus of the Tonner group with applications ranging from transition metal and organic molecular chemistry to surface chemistry.[2-6] Specifically, we developed a powerful bonding analysis method (energy decomposition method for extended systems, pEDA) based on molecular schemes.[1] This analysis allows the breakdown of bonding interactions in physically well-defined terms: electrostatic interaction, Pauli repulsion, and orbital interaction. We complemented this approach by applying a semiempirical dispersion correction scheme (DFT-D3), which has now been adopted by most researchers in the field. We investigated the activation of H2 in main-group systems with DFT and wavefunction-based methods (CCSD(T)), which is a basis for studying DHI activation.[7] Recently, we found H-transfer to be decisive for the surface chemistry of corroles with aromaticity-driven electron transfer, again combining hydrogen-based reactivity and bonding analysis.[8]
This experience will be our starting point to quantify hydrogen isotope effects on chemical bonding.
References
- [1] M. Raupach, R. Tonner, “A periodic energy decomposition analysis method for the investigation of chemical bonding in extended systems“, J. Chem. Phys. 2015, 142, 194105.
- [2] R. Tonner, G. Heydenrych, G. Frenking, “Bonding analysis of n-heterocyclic carbene tautomers and phosphine ligands in transition-metal complexes: A theoretical study“, Chem.Asian J. 2007, 2, 1555-1567.
- [3] J.-N. Luy, S. A. Hauser, A. B. Chaplin, R. Tonner, “Rhodium(I) and iridium(I) complexes of the conformationally rigid ibioxme4 ligand: Computational and experimental studies of unusually tilted nhc coordination geometries“, Organometallics 2015, 34, 5099-5112.
- [4] L. Pecher, R. Tonner, “Deriving bonding concepts for molecules, surfaces, and solids with energy decomposition analysis for extended systems“, WIREs Comput. Mol. Sci. 2019, 9, e1401.
- [5] L. Pecher, S. Laref, M. Raupach, R. Tonner, “Ethers on Si(001): A prime example for the common ground between surface science and molecular organic chemistry“, Angew. Chem. Int. Ed. 2017, 56, 15150-15154.
- [6] A. R. Nodling, R. Möckel, R. Tonner, G. Hilt, “Lewis acids as activators in CBS-catalysed Diels-Alder reactions: Distortion induced Lewis acidity enhancement of SnCl4“, Chem.Eur. J. 2016, 22, 13171-13180.
- [7] M. Rullich, R. Tonner, G. Frenking, “P-heterocyclic carbenes as effective catalysts for the activation of single and multiple bonds. A theoretical study“, New J. Chem. 2010, 34, 1760-1773.
- [8] M. Zugermeier, J. Herritsch, J. N. Luy, M. Chen, B. P. Klein, F. Niefind, P. Schweyen, M. Broring, M. Schmid, R. Tonner, J. M. Gottfried, “On-surface formation of a transient corrole radical and aromaticity-driven interfacial electron transfer“, J. Phys. Chem. C 2020, 124, 13825-13836.
Quantifying hydrogen isotope effects on chemical bonding
The theoretical study of material properties involving nuclear quantum effects (NQE) is challenging, but can be conducted accurately with molecular dynamics (MD). Among MD methods, semi-classical path-integral methods constitute a sweet spot, allowing the description of NQE beyond the computationally efficient classical MD while still being computationally feasible for systems comprising a few dozens of atoms. NQE can be included, for instance, via ring-polymer methods that use replicas of the classical system to obtain nuclear dynamics deviations from the classical paths. However, these methods are seriously limited by high computational efforts associated with quantum chemical calculations of potential energy surfaces (PES) and the many replicas as well as small time steps needed to keep up with rapid fluctuations, which are especially relevant when describing hydrogen atoms. Deep learning (DeepL) can accelerate path integral MD of small molecules by fitting PES, but limitations due to the quantum description of the nuclear propagation still restrict MD studies to small time and length scales.[1] To overcome this problem, we propose to develop a new method based on DeepL to enable the description of NQE with the computational efficiency of classical MD.

Previous work: The PI has pioneered research in DeepL for MD[1,2] and the integration of DeepL into computational workflows.[3] So far, she has enabled the investigation of perturbed systems on experimentally relevant time scales,[4,5] considered infeasible just a few years ago. She has developed physics-inspired DeepL to sample nanoclusters on surfaces,[6] describe molecular adsorption on nanoparticles,[6] and calculate absorption[7] and photoemission spectra with experimental accuracy[8] – topics that are highly relevant for the proposed research. Finally, her group has used a combination of DeepL for high-throughput screening of electronic properties of organic molecules in conjunction with unsupervised generative modelling for property-driven molecular design.[9]
Aim: In P15, the PES, corresponding forces, and dipole moments of dihydrogen isotope (DHI) complexes of M+ and of DHI on nanoparticles (P1) will be learned with deep neural networks at coupled cluster with single, double and perturbed triple excitations (CCSD(T)) accuracy. Data will be sampled using adaptive sampling and active learning protocols.[5] While this approach can be considered state-of-the-art, it goes into directions currently not pursued by employing a second DeepL model to correct every time step in the classical MD to account for NQE. Therefore, the potential differences will be learned at different time steps during the MD (Figure P15). Moreover, Fourier transform of the autocorrelation of the dipole moments obtained from MD will be used to compute infrared spectra with and without NQEs, allowing for direct assessment of the importance of NQE on the MD and spectra. The accuracy of the method will be assessed by comparison to experimentally measured vibrational frequencies and rate constants from P1. Finally, as the DeepL models provide an analytic description of DHI on metal cations and nanoparticles, target properties can be optimised with respect to atomic position and elemental composition, enabling inverse design.[9] The envisioned method will thus not only provide insight into the influence of NQE on RTG-relevant systems, but also guide the design of hydrogen isotope separation materials.
References
- [1] J. Westermayr, P. Marquetand, “Machine learning for electronically excited states of molecules”, Chem. Rev. 2021, 121, 9873–9926.
- [2] J. Westermayr & P. Marquetand, “Machine learning and excited-state molecular dynamics”, Mach. Learn.: Sci. Technol. 2020, 1, 043001.
- [3] B. Lier, P. Poliak, P. Marquetand, J. Westermayr, C. Oostenbrink, “BuRNN: Buffer Region Neural Network approach for polarizable-embedding neural network/molecular mechanics simulations”, J. Phys. Chem. Lett., 2022, 13(17), 3812-3818.
- [4] J. Westermayr, M. Gastegger, D. Vörös, L. Panzenboeck, F. Joerg, L. González, P. Marquetand, “Deep learning study of tyrosine reveals that roaming can lead to photodamage”, Nat. Chem. 2022, 14, 914-919.
- [5] J. Westermayr, M. Gastegger, M. F. S. J. Menger, S. Mai, L. González, P. Marquetand, “Machine learning enables long time scale molecular photodynamics simulations”, Chem. Sci. 2019, 10, 8100-8107.
- [6] J. Westermayr, S. Chaudhuri, S. Jeindl, O. T. Hofmann, R. J. Maurer Maurer, “Long-range dispersion-inclusive machine learning potentials for structure search and optimization of hybrid organic–inorganic interfaces”, Digit. Discov. 2022, 1, 463-475.
- [7] J. Westermayr, P. Marquetand, “Deep learning for UV absorption spectra with SchNarc: First steps toward transferability in chemical compound space”, J. Chem. Phys. 2020, 153, 154112.
- [8] J. Westermayr, R. J. Maurer, “Physically inspired deep learning of molecular excitations and photoemission spectra”. Chem. Sci. 2021, 12, 10755-10764.
- [9] J. Westermayr, J. Gilkes, R. Barrett, R. J. Maurer, “High-throughput property-driven generative design of functional organic molecules”, preprint, arXiv:2207.01476 2022, (accepted in Nat. Comp. Sci.).
Reaction acceleration in charged microdroplets for catalyst-free deuterations
Charged microdroplets provide a special reaction environment, which was found to accelerate various organic reactions by many orders of magnitude.[1,2] These findings open up the possibility to substitute expensive catalysts in chemical reactions by “electrospraying” a solution of the reagent. This method has already been successfully applied to small-scale chemical synthesis. Detailed mechanistic studies on the effect have proven to be challenging due to the large number and variety of parameters involved. Among others, partial solvation of reagents, strong electric fields at the droplet interface and extreme pH values due to an acid-base double layer at the outer rim of the droplet have been discussed.[1,2] Many investigated reactions are acid-catalyzed,[1] but redox reactions, including spontaneous reduction of organic molecules in droplets, have also been reported.[3] Recent insights on the importance of the equilibrium 2 H2O ⇌ H2O•+ + H2O• –[4] provide the basis for current models, which propose a double layer of H2O•+ + H2O• – at the droplet interface. This double layer serves as an explanation for spontaneous redox processes and may allow the acceleration of reactions that would otherwise require alternative electrochemical or photocatalytic activation.
We plan to investigate selective deuteration approaches of organic substrates in charged microdroplets, which are typically driven by redox catalysts.
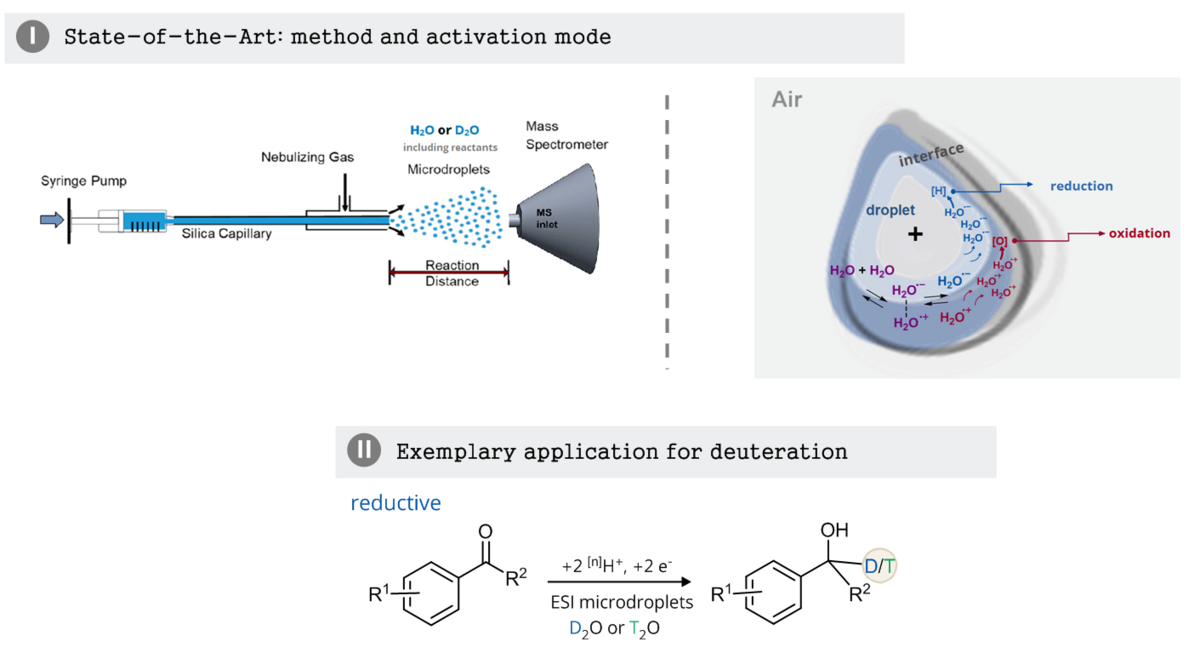
ESI-MS equipment and deuterated solvents will be used to investigate C–D bond formations dependent on various spray conditions. Building on the special conditions in water microdroplets,[5] we will exploit these using D2O, to develop and study selective reductive and oxidative deuteration processes. We will start with simple reactions which are known in the literature to result in C–H bond formation, e.g. the reduction of acetophenone.[3] For mechanistic investigations, our test reagents will be modified and fine-tuned synthetically (Zeitler group), e.g. by providing different functional groups and tags for investigation of their electronic effects on the deuteration or the covalent coupling to pre-charged groups. Optimized reaction conditions will then be transferred to a preparative electrospray deposition setup available in the Warneke group.
The Warneke group is experienced in electrospray ionization (ESI) mass spectrometry for analytical and preparative applications.[6] ESI enables the generation of charged microdroplets coupled to direct mass spectrometric analysis of the reaction products. The group recently contributed to mechanistic studies in the context of reaction acceleration in charged microdroplets.[7] The Zeitler group has a long-term experience in the area of photoredox catalysis, in particular in the investigation of different multicatalytic photochemical and photoredox approaches, including combinations with hydrogen atom transfer (HAT), hydrogen-bond activation,[8] enamine[9] or Lewis acid catalysis.[10]
References
- [1] X. Yan, R. M. Bain, R. G. Cooks, “Organic Reactions in Microdroplets: Reaction Acceleration Revealed by Mass Spectrometry”, Angew. Chem. Int. Ed. 2016, 55, 12960–12972.
- [2] L. Qiu, R. G. Cooks, “Spontaneous Oxidation in Aqueous Microdroplets: Water Radical Cation as Primary Oxidizing Agent”, Angew. Chem. Int. Ed. 2024, 63, e202400118.
- [3] J. K. Lee, D. Samanta, H. G. Nam, R. N. Zare, “Micrometer-Sized Water Droplets Induce Spontaneous Reduction”, J. Am. Chem. Soc. 2019, 141, 10585−10589.
- [4] D. Ben-Amotz, “Electric buzz in a glass of pure water”, Science 2022, 376, 800–801.
- [5] S. Jin, H. Chen, X. Yuan, D. Xing, R. Wang, L. Zhao, D. Zhang, C. Gong, C. Zhu, X. Gao, Y. Chen, X. Zhang, “The Spontaneous Electron-Mediated Redox Processes on Sprayed Water Microdroplets“, JACS Au 2023, 3, 1563−1571.
- [6] M. Rohdenburg, Z. Warneke, H. Knorke, M. Icker, J. Warneke, “Chemical Synthesis with Gaseous Molecular Ions: Harvesting [B12Br11N2]− From a Mass Spectrometer“, Angew. Chem. Int. Ed. 2023, 62, e202308600.
- [7] F. Yang, R. Urban, J. Lorenz, J. Griebel, N. Koohbor, M. Rohdenburg, H. Knorke, D. Fuhrmann, A. Charvat, B. Abel, V. A. Azov, J. Warneke, “Control of Intermediates and Products by Combining Droplet Reactions and Ion Soft-Landing”, Angew. Chem. Int. Ed. 2024, 63, e202314784.
- [8] M. Neumann, K. Zeitler, “A cooperative hydrogen-bond-promoted organophotoredox catalysis strategy for highly diastereoselective, reductive enone cyclization“, Chem. Eur. J. 2013, 19, 6950–6955.
- [9] M. Neumann, S. Füldner, B. König, K. Zeitler, “Metal-free, cooperative asymmetric organophotoredox catalysis with visible light”, Angew. Chem. Int. Ed. 2011, 50, 951–954.
- [10] E. Speckmeier, P. J. W. Fuchs, K. Zeitler, “A synergistic LUMO lowering strategy using Lewis acid catalysis in water to enable photoredox catalytic, functionalizing C‑C cross-coupling of styrenes“, Chem. Sci. 2018, 9, 7096–7103.